Algae, Phytoplankton and Chlorophyll
What are Algae?
Algae are aquatic, plant-like organisms. They encompass a variety of simple structures, from single-celled phytoplankton floating in the water, to large seaweeds (macroalgae) attached to the ocean floor 2. Algae can be found residing in oceans, lakes, rivers, ponds and even in snow, anywhere on Earth.
So what makes algae only plant-like, instead of plants? While algae are often called primitive plants, other terms, like protists, can be used 4. Protist may be a more accurate term, particularly for the single-celled phytoplankton 8. However, larger, more complex algae, including kelp and chara, are often mistaken for submerged plants.
The difference between these seaweeds and submerged plants is in their structure. Macroalgae are simpler, and attach themselves to the seabed with a holdfast instead of true roots 4. Aquatic plants, whether floating, submerged, or emergent (starting in the water and growing out) have specialized parts such as roots, stems and leaves 3. Most plants also have vascular structures (xylem and phloem), which carry nutrients throughout the plant. While algae contain chlorophyll (like plants), they do not have these specialized structures 8.
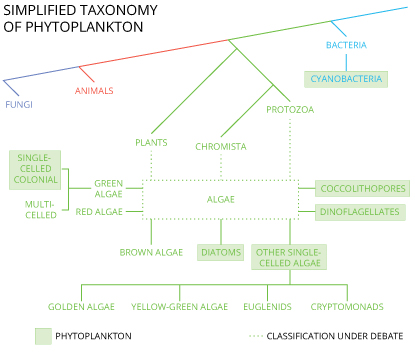
As algae can be single-celled, filamentous (string-like) or plant-like, they are often difficult to classify. Most organizations group algae by their primary color (green, red, or brown), though this creates more problems than it solves 4. The various species of algae are vastly different from each other, not only in pigmentation, but in cellular structure, complexity, and chosen environment 4,5. As such, algal taxonomy is still under debate, with some organizations classifying algae under different kingdoms, including Plantae, Protozoa and Chromista 4,6,8,9. While the overarching kingdom classification is not always agreed upon, the species, genus, family, class and phylum of each alga generally are 6.
To further complicate this nomenclature, single-celled algae often fall under the broad category of phytoplankton.
What are phytoplankton?
Phytoplankton are microorganisms that drift about in water. They are single-celled, but at times they can grow in colonies large enough to be seen by the human eye 16. Phytoplankton are photosynthetic, meaning they have the ability to use sunlight to convert carbon dioxide and water into energy 11. While they are plant-like in this ability, phytoplankton are not plants. The term “single-celled plants” is a misnomer, and should not be used. Instead, phytoplankton can be divided into two classes, algae and cyanobacteria 10. These two classes have the common ability of photosynthesis, but have different physical structures. Regardless of their taxonomy, all phytoplankton contain at least one form of chlorophyll (chlorophyll A) and thus can conduct photosynthesis for energy.
Phytoplankton, both algae and cyanobacteria, can be found in fresh or saltwater 13. As they need light to photosynthesize, phytoplankton in any environment will float near the top of the water, where sunlight reaches 10. Most freshwater phytoplankton are made up of green algae and cyanobacteria, also known as blue-green algae 13. Marine phytoplankton are mainly comprised of microalgae known as dinoflagellates and diatoms, though other algae and cyanobacteria can be present. Dinoflagellates have some autonomous movement due to their “tail” (flagella), but diatoms are at the mercy of the ocean currents 12.
Microalgae
There are thousands of species of planktonic algae, or microalgae, floating in water all over the world. Green algae, diatoms and dinoflagellates are the most well-known, though other microalgae species include coccolithophores, cryptomonads, golden algae, yellow-green algae and euglenoids 1. There are so many diatoms drifting in the oceans that their photosynthetic processes produce about half of Earth’s oxygen 9. While diatoms and dinoflagellates are forms of planktonic algae, they can be incorrectly classified as red or brown algae 9. Red and brown algae are not considered phytoplankton as they are not free-floating. True red and brown algae are rarely single-celled, and remain attached to rock or other structures instead of drifting at the surface 1,17. Multicellular green algae is also not considered phytoplankton for the same reasons. To be considered a phytoplankton, the algae needs to use chlorophyll A in photosynthesis, be single-celled or colonial (a group of single-cells), and live and die floating in the water, not attached to any substrate 1.
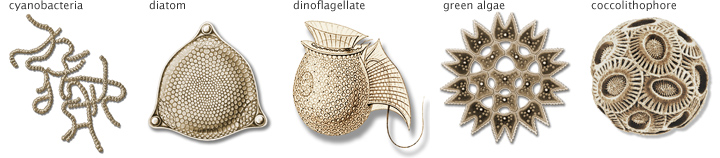
Cyanobacteria: Blue-Green Algae
Despite their ability to conduct photosynthesis for energy, blue-green algae are a type of bacteria. This means that they are single-celled, prokaryotic (simple) organisms. Prokaryotic means that the cyanobacteria do not have a nucleus or other membrane-bound organelles within their cell wall 5.
Cyanobacteria are the only bacteria that contain chlorophyll A, a chemical required for oxygenic photosynthesis (the same process used by plants and algae) 1,14. This process uses carbon dioxide, water and sunlight to produce oxygen and glucose (sugars) for energy. Chlorophyll A is used to capture the energy from sunlight to help this process. Other bacteria can be considered photosynthesizing organisms, but they follow a different process known as bacterial photosynthesis, or anoxygenic photosynthesis 14. This process uses bacteriochlorophyll instead of chlorophyll A 19. These bacteria cells use carbon dioxide and hydrogen sulfide (instead of water) to manufacture sugars. Bacteria cannot use oxygen in photosynthesis, and therefore produce energy anaerobically (without oxygen) 18. Cyanobacteria and other phytoplankton photosynthesize as plants do, and produce the same sugar and oxygen for use in cellular respiration.
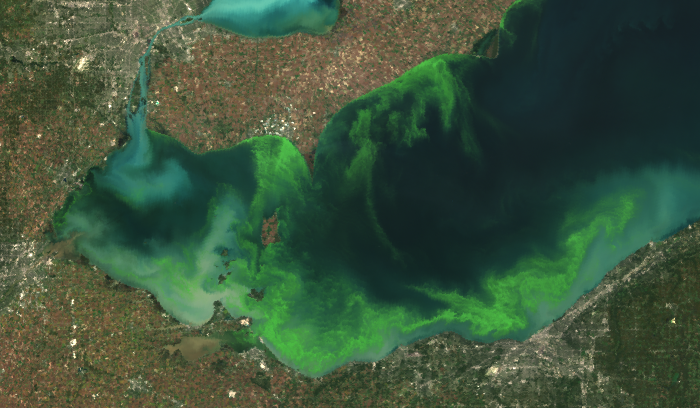
In addition to chlorophyll A, blue-green algae also contain the pigments phycoerythrin and phycocyanin, which give the bacteria their bluish tint (hence the name, blue-green algae) 15. Despite not having a nucleus, these microorganisms do contain an internal sac called a gas vacuole that helps them to float near the surface of the water 13.
What is chlorophyll?
Chlorophyll is a color pigment found in plants, algae and phytoplankton. This molecule is used in photosynthesis, as a photoreceptor 20. Photoreceptors absorb light energy, and chlorophyll specifically absorbs energy from sunlight 15. Chlorophyll makes plants and algae appear green because it reflects the green wavelengths found in sunlight, while absorbing all other colors.
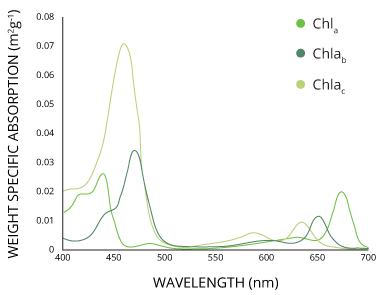
However, chlorophyll is not actually a single molecule. There are 6 different chlorophylls that have been identified 1,22. The different forms (A, B, C, D, E and F) each reflect slightly different ranges of green wavelengths. Chlorophyll A is the primary molecule responsible for photosynthesis 1,15. That means that chlorophyll A is found in every single photosynthesizing organism, from land plants to algae and cyanobacteria 1. The additional chlorophyll forms are accessory pigments, and are associated with different groups of plants and algae and play a role in their taxonomic confusion. These other chlorophylls still absorb sunlight, and thus assist in photosynthesis 20. As accessory pigments, they transfer any energy that they absorb to the primary chlorophyll A instead of directly participating in the process 1,21.
Chlorophyll B is mainly found in land plants, aquatic plants and green algae 1. In most of these organisms, the ratio of chlorophyll A to chlorophyll B is 3:1 21. Due to the presence of this molecule, some organizations will group the green algae into the Plant Kingdom. Chlorophyll C is found in red algae, brown algae, and dinoflagellates 15. This has lead to their classification under the Kingdom Chromista 4. Chlorophyll D is a minor pigment found in some red algae, while the rare Chlorophyll E has been found in yellow-green algae. Chlorophyll F was recently discovered in some cyanobacteria near Australia 22. Each of these accessory pigments will strongly absorb different wavelengths, so their presence makes photosynthesis more efficient 20.
Other Color Pigments
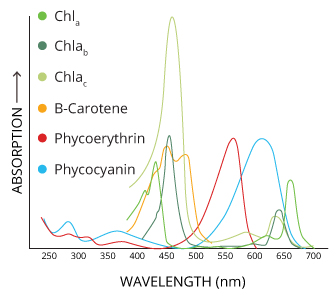
Chlorophyll is not the only photosynthetic pigment found in algae and phytoplankton. There are also carotenoids,and phycobilins (biliproteins). These accessory pigments are responsible for other organism colors, such as yellow, red, blue and brown. Like chlorophylls B, C, D, E and F, these molecules improve light energy absorption, but they are not a primary part of photosynthesis. Carotenoids can be found in nearly every phytoplankton species, and reflect yellow, orange and/or red light 15. There are two phycobilins found in phytoplankton: phycoerythrin and phycocyanin. Phycocyanin reflects blue light and is responsible for cyanobacteria’s common name – blue-green algae. Phycoerythrin reflects red light, and can be found in red algae and cyanobacteria.
Some algae will appear green despite the presence of these accessory pigments. Just as in plants, the chlorophyll in algae has a stronger relative absorption than the other molecules. Like a dominant trait, the more intense, reflected green wavelengths can mask the other, less-reflected colors 20. In green algae, chlorophyll is also found at a higher concentration relative to the accessory pigments. When the accessory pigments are more concentrated (such as in red algae, brown algae and cyanobacteria), the other colors can be seen 23.
What is Photosynthesis?
Photosynthesis is the process by which organisms use sunlight to produce sugars for energy. Plants, algae and cyanobacteria all conduct oxygenic photosynthesis 1,14. That means they require carbon dioxide, water, and sunlight (solar energy is collected by chlorophyll A). Plants and phytoplankton use these three ingredients to produce glucose (sugar) and oxygen. This sugar is used in the metabolic processes of the organism, and the oxygen, produced as a byproduct, is essential to nearly all other life, underwater and on land 1,24.
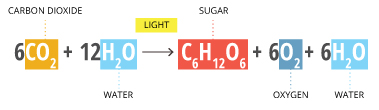
Underwater Photosynthesis
Phytoplankton drifting about below the surface of the water still carry out photosynthesis. This process can occur as long as enough light is available for the chlorophyll and other pigments to absorb. In the ocean, light can reach as far as 200m below the surface 25. This region where sunlight can reach is known as the euphotic zone. Phytoplankton and other algae can be found throughout this zone.
What Affects Photosynthesis?
As light is required for photosynthesis to occur, the amount of light available will affect this process. Photosynthetic production peaks during the day and declines after dark 24. However, not all light can be used for photosynthesis. Only the visible light range (blue to red) is considered photosynthetically active radiation 1. Ultraviolet light has too much energy for photosynthesis, and infrared light does not have enough. If phytoplankton are exposed to too much UV light, the excessive solar energy can break molecular bonds and destroy the organisms’ DNA 27.
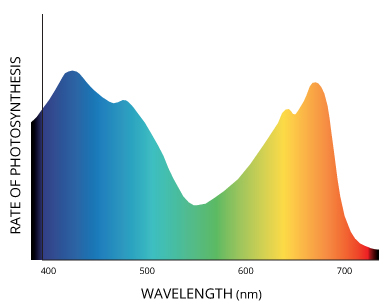
Within the visible light spectrum, chlorophyll strongly absorbs red and blue light while reflecting green light 48. This is why phytoplankton, particularly cyanobacteria, can thrive at the bottom of the euphotic (sunlit) zone, where only blue light can reach. As blue light is both high in energy and strongly absorbed by chlorophyll, it can be used effectively in photosynthesis.
Turbidity, or the presence of suspended particles in the water, affects the amount of light that reaches into the water 1. The more sediment and other particles in the water, the less light will be able to penetrate. With less light available, photosynthetic production will decrease. In turbid water, photosynthesis is more likely to occur at the water’s surface than on the lakebed, as more light is available. .
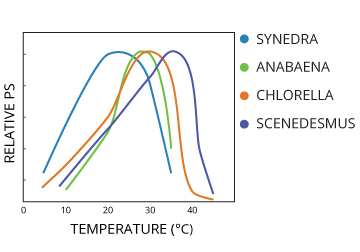
Water temperature will also affect photosynthesis rates 1. As a chemical reaction, photosynthesis is initiated and sped up by heat 26. As photosynthesis production increases, so will phytoplankton reproduction rates 13. This factors into the large, seasonal swings of phytoplankton populations 13. However, the extent to which temperature affects photosynthesis in algae and cyanobacteria is dependent on the species. For all phytoplankton, photosynthetic production will increase with the temperature, though each organism has a slightly different optimum temperature range 1. When this optimum temperature is exceeded, photosynthetic activity will in turn be reduced. Too much heat will denature (break down) the enzymes used during the process, slowing down photosynthesis instead of speeding it up 26.
Why are Phytoplankton Important?
Microscopic phytoplankton play some of the biggest roles in climate control, oxygen supply and food production. These single-celled organisms are responsible for more than 40% of Earth’s photosynthetic production 28. That process uses up carbon dioxide, which helps regulate CO2 levels in the atmosphere, and produces oxygen for other organisms to live 28.
Oceanic Food Web
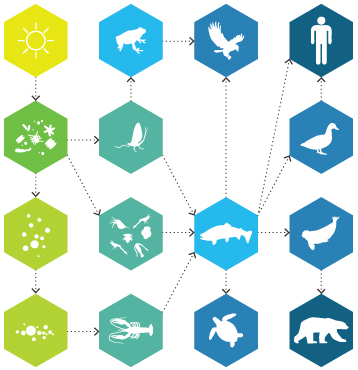
Phytoplankton make up the foundation of the oceanic food web. A food web is a complex net of organisms and food chains (who-eats-who). To survive, every living thing needs organic carbon 29. Organic carbon can be found in many different things including sugars (glucose = C6H12O6), plants and animals. Phytoplankton produce their required sugar through photosynthesis. As they are able to produce their own energy with the help of light, they are considered autotrophic (self-feeding). Phytoplankton and other autotrophs are called primary producers, and make up the bottom of the food web 11. These organisms are called “primary” because all other organisms rely on them (directly or indirectly) as a food source 29.
Phytoplankton are generally consumed by zooplankton and small marine organisms like krill. These creatures are then consumed by larger marine organisms, such as fish 29,30. This chain continues up to apex predators, including sharks, polar bears and humans.
Oxygen Production
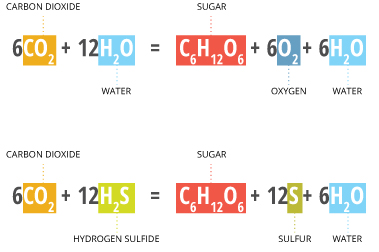
During the photosynthetic process, phytoplankton produce oxygen as a byproduct. Due to their vast and widespread populations, algae and cyanobacteria are responsible for approximately half of all the oxygen found in the ocean and in our atmosphere 10. Thus oceanic lifeforms not only feed off the phytoplankton, but also require the dissolved oxygen they produce to live.
Before plants, algae and phytoplankton used water for photosynthesis, bacteria used H2S and other organic compounds to fix CO2 31. Early cyanobacteria were the first organism to use water to fix carbon 31. The use of H2O introduced free oxygen (O2) into the environment as a byproduct. The start of oxygenic photosynthesis was a turning point for Earth’s history. This process slowly changed the inert Precambrian atmosphere into the oxygen-rich environment known today 31. Though microscopic, early cyanobacteria have made a permanent impact on the Earth’s environment.
Carbon Fixation and the Climate
In addition to providing food and oxygen for nearly all life on Earth, phytoplankton help to regulate inorganic carbon (carbon dioxide) in the atmosphere 17. During photosynthesis, carbon dioxide and water molecules are used to make sugar for energy. The process of incorporating inorganic carbon into organic carbon (glucose and other biologically useful compounds) is called carbon fixation, and is part of the biological carbon pump 11.
As carbon fixation and oxygen production are part of the same process, the extent of phytoplankton’s participation is on the same scale. Phytoplankton consume a similar amount of carbon dioxide as all land plants combined 11. While phytoplankton can pull carbon dioxide from the atmosphere or the ocean, it will have a similar effect. CO2 that is taken from the water is replaced by CO2 from the atmosphere, thanks to Henry’s law (the dissolved gas content of water is proportional to the percentage of gas in the air above it 32. This consumption helps keep carbon dioxide levels in check, reducing its presence as a greenhouse gas 28.
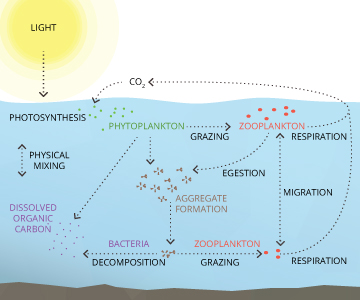
When carbon dioxide is consumed, the carbon molecules become incorporated into the phytoplankton’s structure, allowing the organism to function and grow 11. If the phytoplankton is not eaten by another organism (passing on the carbon up the food chain), then it will sink into the ocean when it dies. As with other detritus (non-living organic material), the phytoplankton will be decomposed by bacteria, and the carbon is either released back into the ocean as dissolved carbon dioxide or eventually deposited into the seafloor sediment 33. Thanks to phytoplankton, this biological carbon pump removes approximately 10 trillion kilograms (10 gigatonnes) of carbon from the atmosphere every year, transferring it to the ocean depths 11.
In climate terms, this process helps to maintain global surface temperatures 11. Without this cycle, atmospheric CO2 would rise approximately 200 ppm (current levels are around 400 ppm) 33,34. Even small changes in phytoplankton populations could have an effect on the atmosphere and world climate 11.
Typical Levels and Factors that Influence Productivity
Phytoplankton populations and their subsequent photosynthetic productivity will fluctuate due to a number of factors, most of which are part of seasonal changes 30. The largest influence on phytoplankton levels is nutrient scarcity 13. While sunlight levels affect productivity, nutrient levels affect phytoplankton growth and populations. While any one phytoplankton only lives for a few days, a population boom can last for weeks under the right conditions 11.
As phytoplankton populations grow and shrink seasonally, typical concentrations vary not only by location but from month to month 30. Expected levels should be based on local, seasonal data from previous years. While changes within the same calendar year are normal, populations should stay consistent with previous seasonal fluctuations from year to year. If phytoplankton concentrations are abnormally high or low for a season, it may indicate other water quality concerns that should be addressed.
Sunlight Influence
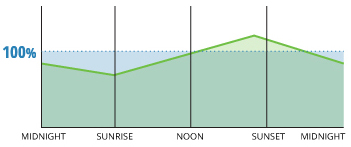
Phytoplankton require sunlight for photosynthesis. If sunlight is limited, phytoplankton productivity will decrease. This can be seen in a daily cycle as oxygen levels fluctuate with light levels throughout the day. However, if sunlight is unavailable or minimal for an extended period of time, aquatic life will consume dissolved oxygen quicker than phytoplankton can restore it, leading to a plummet in dissolved oxygen levels 1. Phytoplankton are responsible for much of the dissolved oxygen found in surface waters 10. As oxygen is required for fish and other aquatic organisms, a decrease in photosynthesis productivity is detrimental to aquatic populations. Without phytoplankton, the oxygen supply of the ocean would be cut in half. In both fresh and saltwater, a lengthy decrease in phytoplanktonic productivity can lead to a fish kill (massive fish die-off) 1.
Although phytoplankton require sunlight for photosynthesis and oxygen production, too much light can be harmful to photosynthetic production. Ultraviolet light from the sun can damage the phytoplanktons’ DNA, inhibiting the photosynthetic pathway 35. On very bright days, UV-B radiation can diminish photosynthesis by 8.2% 35. This is why photosynthesis rates peak during the morning, and decrease at noon (when the radiation levels are highest) 1.
Nutrient Influence
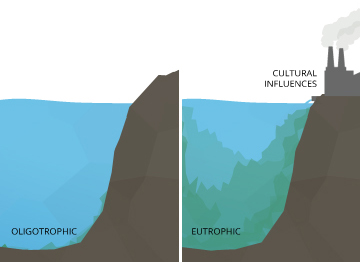
While phytoplankton rely on photosynthesis to produce sugar for energy, they still need other nutrients to grow and reproduce 7. These nutrients are typically phosphorus, nitrogen and iron, though some species also require silicon, calcium and other trace metals 11,13. The more nutrients (particularly phosphorus) that are present in a body of water, the more algae and phytoplankton that will grow 7. An increase in the nutrient concentration of a body of water is called eutrophication 13. Eutrophication is often an indicator of agricultural runoff, which can raise phosphorus and nitrogen concentrations to very high levels. If there are too many nutrients, the algae will form a bloom, which can be very detrimental to water quality and aquatic health 7.
The lack of iron in the open ocean limits phytoplankton growth 10. Nitrogen and phosphorus are also scarce away from coastlines, and can be limiting factors as well 13. However, ocean circulation can cause an upwelling, which moves deep, nutrient-rich water up into the photic (sunlight zone), replacing the nutrient-depleted surface water 30. Upwelling, seasonal ice melts and agricultural runoff can all increase nutrient levels, leading to an increase in phytoplankton populations.
Typical Freshwater Levels
In temperate fresh waters, growth is limited in winter because light and temperatures are low. A large increase in the spring normally occurs as light conditions improve and water begins to mix 1. In the summer, phytoplankton flourish until the nutrient supply begins to run low. In tropical lakes, the phytoplankton distribution is fairly constant throughout the year and seasonal population changes are often very small 1. In temperate and subpolar waters, the seasonal fluctuations are normally fairly large. Fluctuations in population also occur if agricultural runoff brings additional nutrients into a body of water.
Typical Saltwater Levels
Saltwater phytoplankton can be found all over the world, living in the photic (sunlit zone) of the ocean. Cyanobacteria prefer to live near the bottom of this zone, closest to the nutrient-rich deep water while still receiving enough sunlight for photosynthesis 1. However, in any marine environment, phytoplankton populations vary not only by season but by region.
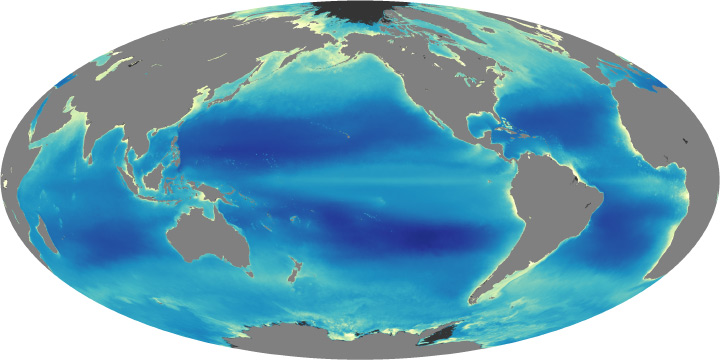
Algae blooms can occur near the poles in the spring, when there is plenty of sunlight and the melting sea ice leaves behind nutrient-rich freshwater 30. This melting process also fuels the oceanic convection, or circulation 38. In coastal and open-ocean environments, oceanic circulation is responsible for phytoplankton concentrations.
This circulation can cause upwelling (bringing nutrient-rich water to the surface) and instigates phytoplankton transportation. Like sea ice melting, upwelling is a seasonal occurrence. The extent and location of upwells are based on wind patterns, which cause currents across the globe 11. Surface water is carried away from coastlines by currents, and is replaced by cold, nutrient-rich water from below 37.
In many coastal regions, southerly winds cause this coastal upwelling in late summer and autumn 36. As upwelling brings nutrient-rich water up to the surface, phytoplankton blooms often appear at this time. Oceanic circulation and upwelling ensures that the coastal environments have the highest rates of primary production in the ocean 13. Tides, flooding and currents all encourage higher nutrient levels in the photic zone 13.
Consequences of Unusual Levels
Phytoplankton are an important aspect of a healthy body of water. Algae and cyanobacteria help to provide oxygen and food for aquatic organisms 12. As a key component, an imbalance of phytoplankton levels can cause major problems. If too many nutrients are available, it can trigger an algal bloom 12. Algal blooms and overproduction of phytoplankton can cause toxic red tides and fish kills. On the other hand, phytoplanktonic productivity can be limited by a lack of required reactants such as sunlight. This decrease in productivity can also lead to fish kills 3.
Algal Blooms and Red Tides
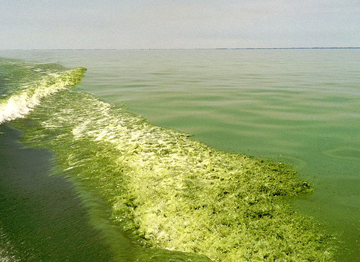
An algal bloom is a sudden increase in the concentration of phytoplankton. During a bloom, clear water can become covered with phytoplankton within days 39. These algal blooms can grow large enough to be seen from a satellite, covering hundreds of square kilometers 11. Algal blooms come in many colors from green to red, brown, blue, white or purple 43.
Under the right conditions, algal blooms can last one week to an entire summer, despite the short, few-day life span of phytoplankton 11. A single bloom will only last one to two weeks, as the phytoplankton population will die without the proper oxygen and nutrient levels. However, if the water conditions stay favorable, successive blooms can occur and appear to be one continuous population 39. Algal blooms are most common in late summer and early fall.
What Causes an Algal Bloom?
There are several causes that can contribute to an algal bloom. These blooms can occur seasonally, after an upwelling of nutrient-rich water, or due to pollution such as agricultural runoff. In both cases, the water becomes saturated with nutrients, creating an ideal environment for phytoplankton productivity 36. Even natural causes can trigger an algal bloom, such as a rainstorm followed by warm, sunny weather 1. Rain can contribute runoff, or encourage the mixing of nutrient-depleted and nutrient-rich layers of water. When nutrient levels rise, phytoplankton growth is no longer nutrient-limited and a bloom may occur 13.
Red Tides
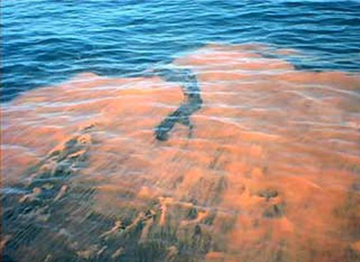
If a phytoplankton concentration stays steady after the initial bloom, it may become a red tide. While some blooms are harmless, others may produce toxins that endanger aquatic life and humans. This harmful algal bloom is known as a red tide. While red tides specifically refer to harmful algal blooms (HABs), they are often simply associated with the discoloration due to a large concentration of phytoplankton 53,43. Although known as a red tide, the discoloration from a harmful algal bloom is not always red. The color of the tide depends on the pigments present in the phytoplankton 36. In some cases, the bloom cannot be seen by the human eye, though it is still releasing toxins 43.
Red tides and the toxins they release can have a direct or indirect impact on the health of humans and other organisms. Some species of phytoplankton can suffocate fish during a bloom by clogging or irritating the fishes’ gills, preventing them from taking in oxygen 53. These harmful algal blooms can also cause shellfish poisoning in humans and other adverse effects 13. Even during non-toxic algal blooms, the aquatic environment can be compromised. Massive levels of phytoplankton respiration and decomposition can reduce dissolved oxygen to unsustainable levels, resulting in the deaths of other aquatic creatures 13.
Toxins
The phytoplankton that cause a red tide are usually comprised of dinoflagellates, diatoms or cyanobacteria. Certain species of these phytoplankton can contain harmful toxins that can affect humans and other animals. At normal levels, heterotrophic bacteria in the water break down the toxins in these organisms before they can become dangerous 51. When an algal bloom appears, the concentration of toxins increases faster than the bacteria can break it down.
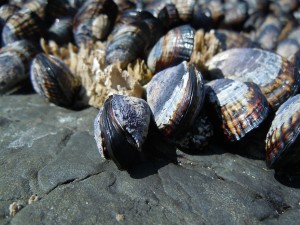
Some of these toxins cause mild problems if consumed by humans, such as headaches and upset stomachs, while others can cause serious neurological and hepatic symptoms that can lead to death 51. These effects can be caused by direct or indirect contact with an algal bloom. Direct exposure can occur from swimming or drinking affected water. Indirect contact can occur from eating animals that have been exposed to the toxic bloom, particularly shellfish.
Shellfish are susceptible to toxins because they are filter feeders. Filter feeders ingest food by taking up the water surrounding them and then filtering out what they do not wish to ingest 52. This method accumulates toxins inside the shellfish system. Organisms that eat the shellfish (including humans) are consuming the concentrated toxins, which can reach deadly levels 52.
Filamentous Algal Bloom
Filamentous algae is a collection of microscopic algae that clumps together in strings and mats at the surface of the water 7. These accumulations can vary from a small, woolly patch near shore to a widespread, slimy green covering. Filamentous algae are often referred to as pond scum, and appear in eutrophic (nutrient-rich) bodies of water. More often than not, filamentous algae are more of a nuisance than a danger 7. They are somewhat more controllable in that the algae clumps can be physically removed from the water 7,44. While large filamentous algal blooms will stop sunlight from penetrating the water and reaching submerged plants, the biggest threat associated with them is oxygen depletion 44.
Oxygen Depletion and Fish Kills
If an algal bloom appears, a fish kill can occur shortly thereafter due to the environmental stresses caused by the bloom. A fish kill, also known as a fish die-off is when a large concentration of fish die. The most common cause of this event is lack of oxygen 45.
If a phytoplankton population grows to an excessive amount, the amount of usable oxygen in the water can be depleted 45. Oxygen depletion has two algal-bloom-related causes: respiration and decomposition. Algae and cyanobacteria consume oxygen at night (respiration) when there is not light for photosynthesis 44. If there is a bloom, the phytoplankton and other aquatic organisms (like fish) can consume more oxygen than is produced. Likewise, if large portions of the algal bloom die off at once, bacteria will start to consume oxygen in order to decompose the dead algae. This can reduce oxygen concentrations to below sustainable levels. If oxygen levels get too low, fish and other aquatic creatures may die 44.
How do you Measure Phytoplankton?
While phytoplankton concentrations can be measured by sampling, this can be difficult and time-consuming. Plankton nets do not always catch the smallest of phytoplankton, and do not provide an accurate estimate of water volume 40. Box or tube traps offer an exact volume, but require lab sedimentation or settling chambers to concentrate the algae population for counting 41. Furthermore, phytoplankton can be found at multiple depths in the water column, which requires multiple sampling efforts and risks missing layers of phytoplankton in between sample depths 40. The main advantage of sampling phytoplankton is the ability to analyze and identify the species present 41.
Measuring Chlorophyll
An easier and more efficient method is to use a chlorophyll sensor. As all phytoplankton have chlorophyll A, a chlorophyll sensor can be used to detect these organisms in-situ 41. In addition to providing immediate data, it can be used for continuous or long-term monitoring and recording. However, as a chlorophyll sensor assumes all algae and cyanobacteria have the same levels of chlorophyll A, it only provides a rough estimate of biomass 41. It also cannot be used to identify specific species.
Even with its limitations, in-situ chlorophyll measurements are recommended in Standard Methods for the Examination of Water and Wastewater to estimate algal populations 32. Chlorophyll sensors are also an in-situ method for determining the trophic state (nutrient-rich, stable, or nutrient-poor) of an aquatic system 47. A high chlorophyll measurement is an indicator of eutrophication.
Chlorophyll is measured in micrograms per liter (µg/l). Chlorophyll sensors rely on fluorescence to estimate phytoplankton levels based on chlorophyll concentrations in a sample of water 47. Fluorescence means that when the chlorophyll is exposed to a high-energy wavelength (approximately 470 nm), it emits a lower energy light (650-700 nm) 47. This returned light can then be measured to determine how much chlorophyll is in the water, which in turn estimates the phytoplankton concentration. These estimates are then used to develop parameter limits for bodies of water. As an example, the New Hampshire Department of Environmental Services provides the following chlorophyll guidelines for river quality: a chlorophyll measurement below 7 µg/l is within a desirable range. 7-15 µg/l is less than desirable, while over 15 µg/l is considered problematic 42.
Measuring Blue-Green Algae
Blue-green algae, or cyanobacteria, are the only phytoplankton that contain phycocyanin and phycoerythrin, making the pigments good indicators of the amount of cyanobacteria in a body of water 15. While chlorophyll measurements can be used to estimate entire phytoplankton populations en masse, the accessory pigments phycocyanin and phycoerythrin can be measured to estimate cyanobacteria concentrations specifically. Marine cyanobacteria have higher levels of phycoerythrin, while freshwater species have dominating amounts of phycocyanin.
Like chlorophyll sensors, blue-green algae sensors rely on fluorescence to detect the pigment concentration 49. Phycoerythrin sensors use a wavelength around 540 nm, while phycocyanin sensors emit a wavelength at 600 nm 50. Due to the differences in secondary pigment concentrations between species, it is recommended to use the phycocyanin BGA sensor in freshwater applications, and the phycoerythrin BGA sensor in saltwater 49,50.
Cite This Work
Fondriest Environmental, Inc. “Algae, Phytoplankton and Chlorophyll.” Fundamentals of Environmental Measurements. 22 Oct. 2014. Web. < https://www.fondriest.com/environmental-measurements/parameters/water-quality/algae-phytoplankton-and-chlorophyll>.
Additional Information
- Measurement Methods
- Chlorophyll Sensors
- Applications
- References